The year 2015 saw the dawn of a new messenger from the cosmos: gravitational waves (GWs). This accelerated the further development of GW detectors on the ground. However, these can only measure GWs of wavelength of order 10-103 km. One needs to go to space for detecting GWs of (much) larger wavelength of which there should be plenty. The Laser Interferometer Space Antenna (LISA) intends to do just that. LISA is the third large mission (L3) of ESA’s Cosmic Vision programme. It is scheduled for launch in the mid-2030s. By being space-borne, the interferometer arms can be much larger than the size of the Earth. LISA will be sensitive to GWs with wavelengths between roughly one million and one billion km. This will open up a huge discovery space, for instance on the enigmatic largest black holes in the universe (supermassive black holes or SMBHs at the nuclei of galaxies) and a phase of the Big Bang much earlier than observable through electromagnetic radiation.
Update: LISA’s mission has been formally adopted and accepted by ESA. Read more in this press release from 25th of January 2024: Zwaartekrachtgolfdetector LISA officieel aangenomen als ESA-ruimtemissie – SRON Netherlands Institute for Space Research
Science
LISA will focus on the following areas of interest:
• Astrophysical black holes. LISA will probe massive black holes over a wide, almost unexplored, range of cosmological redshift (and thus age) and mass, covering essentially all important epochs of their evolutionary history. This touches on the important question of how SMBHs come to be – are they growing from low-mass seeds in the early universe or were the seeds already very massive? It would be a bonus, if the merge of two SMBHs would be detected like the mergers of stellar-sized black holes commonly detected with ground-based GW detectors.

• Extreme mass-ratio inspirals. SMBHs forming galactic nuclei are surrounded by numerous objects of much smaller mass. This may lead to extreme-mass-ratio inspirals and offers, if those smaller objects are compact objects (stellar black holes, neutron stars and white dwarfs), the opportunity to probe the mass and spins of SMBHs between 100 thousand and 10 million solar masses. The detection will unearth such black holes, even when they’re not detectable in electromagnetic radiation, and provide a census of SMBH masses.
• Testing General Relativity. LISA will be able to detect inspirals of stellar-sized black holes and neutron stars one year before the merger and test GR at the limit of high velocities, in other words very strong spacetime deformations at very high signal-to-noise ratio, complementing the measurements with ground-based detectors of the actual merge event.
• The dawn of the universe. LISA probes GW wavelengths that are commensurate with the size of the universe between 10-17 and 10-10 s. GWs emitted at that time thus provide a probe of the early universe, when first order phase transitions (i.e., decoupling of electroweak and weak nuclear forces), supersymmetry and extra dimensions play a strong role. In comparison, EM radiation can only probe the universe after its 300 thousandth birthday and neutrinos after 1 s.
• Ultracompact binaries. These are binaries consisting of a compact object orbiting another star at a distance of at most 106 km with a period of at most 1.5 hr. This period overlaps the LISA frequency band and the detector will be able to substantially increase the known population by many orders of magnitude to several tens of thousands, a rich source for understanding evolution of binaries and direct link to type Ia supernovae. Particularly interesting are the ones that can also be detected in electromagnetic radiation and those that host heavy black holes, such as the LIGO/Virgo prototype source GW150914, that may first be detected by LISA and several years later by the Einstein Telescope. Presumably there are millions of ultracompact binaries that will incur a stochastic GW foreground in LISA.
The Dutch astronomical and physics community has embraced the GW revolution because it has strong connections to gamma-ray bursts, ultracompact binaries, supermassive black holes and the early universe cosmology, and General Relativity.
Mission
LISA is basically a Michelson interferometer with three arms in a closed loop. It uses 1064 nm laser light and three end stations with free-falling test masses only influenced by gravity. The distances between the test masses are measured with picometer accuracy. If there is a residual change after accounting for Keplerian motion, solar wind pressure etc., this will be due to passing GWs and the amplitude and wavelength are measured. All passing GWs are measured simultaneously, with some non-isotropic sensitivity across the sky as a function of azimuthal angle dependence with respect to the plane formed by the three arms of LISA. As LISA’s plane revolves around the Sun on one year, this non-isotropy will cancel out over that time scale.
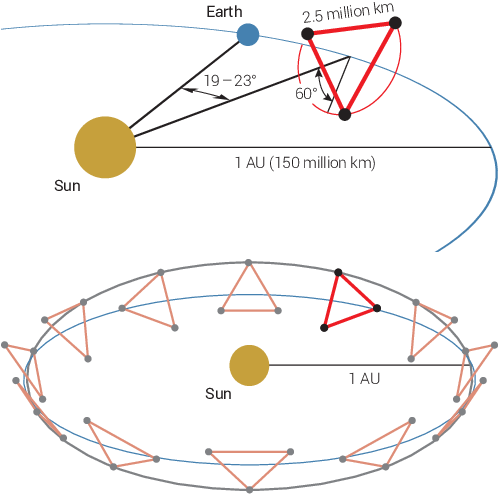
LISA employs a 2.5 million km arm length, which is accomplished by placing three identical spacecraft in a unilateral triangle with 2.5 million km sides. As LISA is so large, it needs to be at a fairly large distance from the Earth and Moon to avoid their gravitational disturbances. Therefore, after launch LISA will cruise to a position 50-60 million km behind Earth in the same orbit. It will take approximately 1.5 years to reach that position. Once there, an operational phase of at least 6 years will commence. The aim is to have significant overlap in the operational life between the Athena X-ray observatory (ESA’s L2 mission) and LISA in order for Athena to be able to follow up interesting detections of LISA.
The three spacecraft are free flying; there is no active formation flying. As the spacecraft are in different orbits, the inter-spacecraft distances will change by about 104 km and the 2 other spacecraft as seen from 1 spacecraft will wobble by about a degree. In fact this angle will change significantly during the light travel time from one spacecraft to the other. The changing distance implies that LISA is a heterodyne interferometer where the interference signal changes with a frequency between 5 and 25 MHz. The lengths of the 3 interferometer arms are measured at picometer accuracy through post-measurement processing in an algorithm called Time Delay Interferometry. Variations in these lengths, after correcting for all kinds of disturbances, are due to GWs.
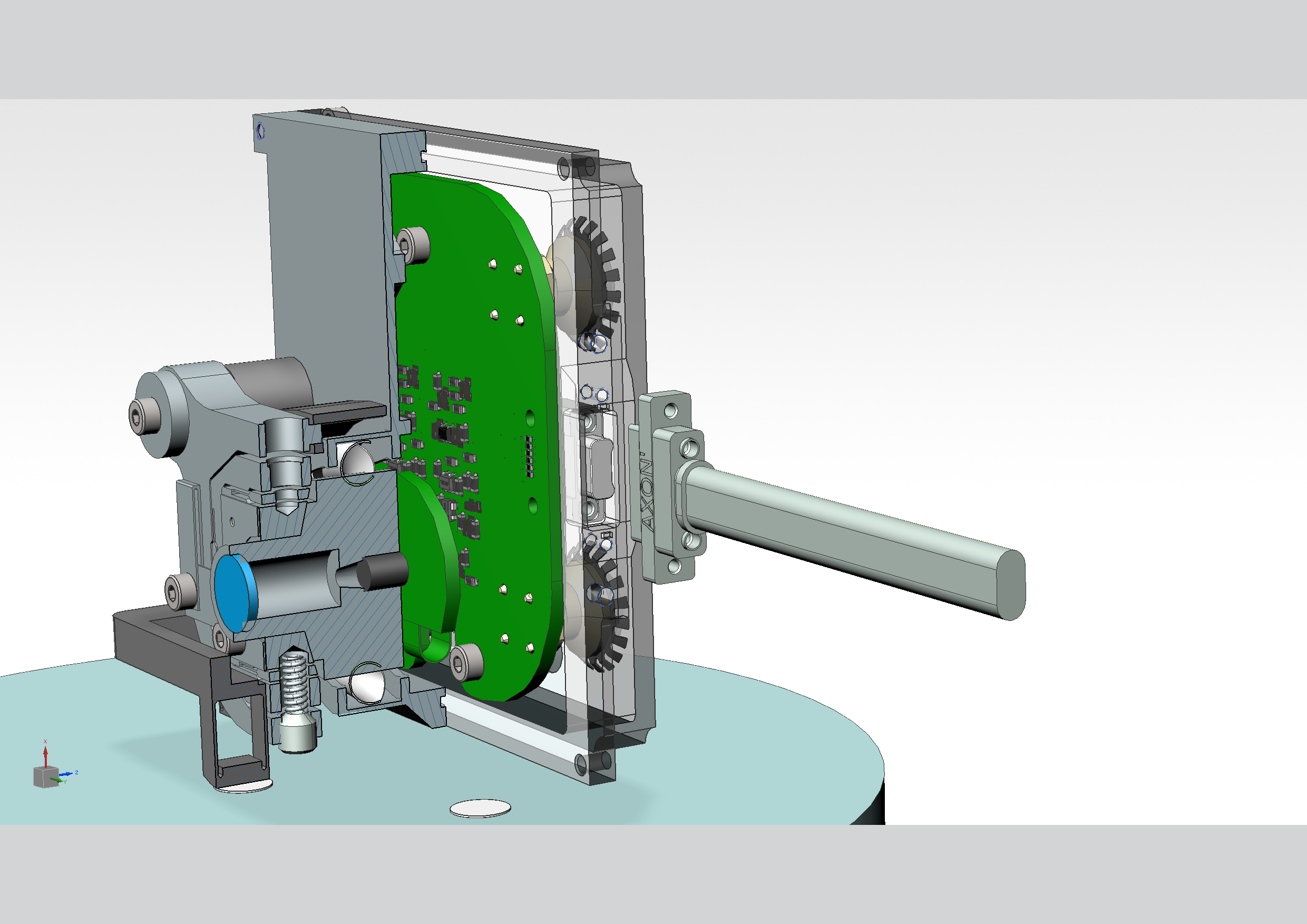
Technology
Each of the three identical spacecraft has two optical benches looking at the two other spacecraft. Each optical bench contains various kinds of components, such as a telescope, lasers, transponders, optical mechanisms and photoreceivers. SRON is involved in the latter two. The photoreceiver (PR) measures the interfering light of two laser beams. Since the laser power in the long-arm interferometer (one of the three interferometers applied) is foreseen to be 0.2 mW (DC) and 0.07% peak-to-peak (AC), the photoreceiver needs to reach a very low noise level of 2 pA/sqrt(Hz) to reach required sensitivity. Furthermore, the laser beam width on the optical bench is aimed to be 2 mm and the photoreceiver needs to be large enough to collect the full beam. As LISA deals with heterodyne interference, the photoreceiver needs to sample the laser light amplitude with a frequency of 5-25 MHz. SRON, in collaboration with Nikhef and Dutch photonics industry (Bright Photonics and Smart Photonics), is developing a new photodiode device to reach the combination of low-noise, large diameter and large dynamic range, and designing a housing for this photodiode and its readout electronics (developed elsewhere) that should adhere to strict thermal requirements to reach a sub-micron stability.
The second contribution from SRON concerns the LISA mechanisms control unit (MCU) containing the ‘front-end’ electronics for the readout and control of the optical mechanisms on the optical bench. Three such mechanisms are part of this: the Point-Ahead Angle Mechanisms (which makes sure the laser beam is pointed accurately enough to the other spacecraft), the Beam Adjustment Mechanism (which makes sure the telescope pupil is accurately positioned on the optical bench) and the Fibre switching Unit (which allows to guide the laser light to one of two redundant optical fibres). SRON will also design the control electronics for the PAAM and lead the integration of the electronics of all three mechanisms in one housing per optical bench. SRON collaborates with TNO and institutes in Czechia and Belgium.

SRON’s contributions to LISA are ultimately dependent on funding from national agencies. Seed funding for the photoreceiver project was, between 2018 and 2021, funded by institutional funds from SRON and Nikhef and by the Nationale Wetenschapsagenda. The development during LISA phase B (until 2024) is funded through an NWO-Midden investment grant. An NWO National Roadmap for Large Scale Facilities was granted in 2023 for further activities for both PR and MCU.